In this blog, we have highlighted how stellar activity can hinder and even masquerade as planetary signals (see Gliese 581 and Gliese 667C). The stars discussed in those posts were important, not only because they may harbor habitable zone planets, but also because they are M Dwarfs. HPF is explicitly being built to discover and confirm planets just like the ones discussed around these stars. There are definitely challenges to overcome, including being able to separate the stellar signals from the planetary signals, however HPF’s use of the near-infrared should help with these problems.

False color image of a solar prominence as seen by NASA’s SDO mission. They have many more images and a fantastic youtube page.
The NIR has a number of advantages when it comes to stellar noise. One of the biggest is that because sunspots are cooler, their emission peak (think of this as a “temperature color.”) is located in or close to the NIR. This reduces the contrast between the normal surface (the photosphere) and the spot areas. We ‘see’ less of a difference between the two areas. Additionally, the effects of high energy events such as solar flares and coronal mass ejections is lessened as they emit much if not most of their light in the visible, ultraviolet, and X-rays.
In addition to its primary mission of searching for habitable planets around M stars, the reduction of stellar noise in the NIR could potentially enable a second exciting exoplanet study with HPF. Today, astronomers are searching for Earth 2.0. We describe this ideal planet as an Earth-sized planet, orbiting a Sun-like star, within the habitable zone. The challenges in finding our twin are immense. Currently we are only sensitive to large planets and planets at shorter periods. To put some numbers to it, the best instruments in the world can currently measure a Doppler shift of ~1 m/s (walking speed). Just as a side, remember, we are doing this across the galaxy! But back to Earth 2.0. If you were an alien observing the Sun, the largest signal you would see is from our most massive planet, Jupiter. Jupiter tugs on the Sun, making it move at about 12 m/s (the top speed of Usain Bolt – the world’s fastest man). The Earth induces a mere 0.1 m/s on the Sun’s velocity (about the speed of a turtle walking on land). This is a TINY number and it is hidden in the signal of other planets and the stellar noise previously discussed!
Since we expect a reduction in the noise around M-Dwarfs when using HPF, the question arose: “could HPF also observe solar type stars and confirm low-mass planets due to a reduction in stellar noise?” Or in other words, could it help discover Earth 2.0? This is actually a complicated question as the mechanism that creates the magnetic activity in M-Dwarfs can be much different from solar activity.
In an attempt to provide an answer, members of the HPF team–led by Penn State graduate student Robert Marchwinski–conducted a study of radial velocity (RV) variations of the Sun as a function of wavelength. In reality, this is a very complicated measurement to make. We are actually too close to the Sun to just point a telescope at it! To do this correctly, we need the Sun to appear unresolved (like a point source). Some ambitious astronomers have tried to do this using reflected light from asteroids in the solar system. However, this introduces many additional factors you have to correct for: like the speed of the asteroid, how it is rotating, its orientation, and more. Thankfully, we have another way.
The F/F’ Technique
Astronomers have long been aware of the problem of stellar activity and much of the research has focused on modeling how activity works and how it would affect measurements. In 2012, a new approach was developed. Dr. Suzanne Aigrain and her collaborators came up with a method to use the brightness variations of a star to estimate the radial velocity variations induced by magnetic activity. This technique is called the F/F’ method. At its core, this method assumes that we have one spot or spotted region that is carried along as the star rotates. Spots emit less light and thus you lose some of the light you would normally see from the region where the spot inhabits. This missing light has an intrinsic Doppler shift thanks to the rotation of the star and the convection of the star’s surface, all of which lost. Thus you can get a slight change in your radial velocity. (See below).

A rotating starspot and its effect on the shape of a stellar absorption line. (Animation credit: Svetlana Berdyugina)
Using this model and combining it with high quality, well-sampled photometry (brightness measurements), you can estimate the Doppler variations you would see coming from a star. We call it the F/F’ method because it uses the flux measurements, F, and the change or derivative of the flux with time, F’ (pronounced “F-prime”).
With this method, some well-educated assumptions, and solar data, we set out to measure these variations as a function of wavelength.
SORCE
Obtaining spectra of stars is normally a time intensive process and you need to have lots of data for this method to work. Thankfully, the Sun is the most well-studied star in the Universe. We use solar measurements to educate us on how stars work, how they are powered, and the effects they have on planetary companions.
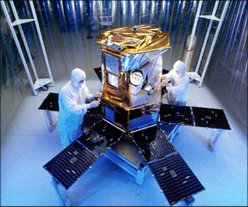
SORCE Satellite in a clean room. Courtesy of LASP.
Enter SORCE. The SOlar Radiation and Climate Experiment was launched by NASA in 2003 and has been observing the Sun almost continuously. The main goal of SORCE is to measure how the variations in solar output affect the climate of the Earth. To do this, it includes an instrument that takes photometric measurements of the Sun, at different wavelengths, everyday. Exactly what we needed! We also have gotten around the issue of needing to see the Sun as a point source. Photometry is much easier to combine and get a disk totaled value then adding spectra together.
With this data in hand, we could then use the F/F’ method to estimate how the RV variations from the Sun change as you look in different wavelength regions.
Results
We found that by looking at solar type stars in the NIR (where HPF will look), you could see a reduction in the stellar magnetic noise by up to a factor of 4! This particular result came from the analysis of solar data over the entire SORCE mission. If you focus on smaller chunks of time, you consistently see that the NIR has lower variability than other regions of the spectrum. While HPF will not have the sensitivity to detect eta-Earth, there is a very good chance that HPF could help detect other low-mass planets and could be extremely useful in identifying signals that are stellar in origin.

The main results from our analysis showing the estimated RV variability of the Sun with wavelength. We have identified the regions where HPF and 2 other optical instruments operate/will operate. The gray region is blocked out because the detector on SORCE introduces noise that we could not correct for.
An additional result was that while the NIR is quieter overall, there are times in the solar cycle where the optical is almost as quiet as the NIR. This happens during the minimum of the solar cycle, when the Sun is the most inactive and quiet. This suggests that if you are looking for eta-Earth and you have knowledge of the target you are looking at, you could optimize your search by looking only during the star’s stellar cycle minimum.
All of these results were extremely promising and we hope to continue building on these results and making the F/F’ method more accurate. For more information, the results will soon be published in the Astrophysical Journal in a paper submitted by team members Robert Marchwinski, Suvrath Mahadevan, Paul Robertson, Larry Ramsey, and collaborator Jerry Harder.