Artist’s rendering of the Neptune-mass exoplanet orbiting the very low-mass star LHS 3154. Video Credit: Abigail Minnich
Introduction
As the name implies, the Habitable-zone Planet Finder was designed primarily to search for Earthlike planets orbiting nearby stars. Its sensitivity to near-infrared light allows us to search for planets around the smallest, coolest stars in the Milky Way Galaxy. There is still a lot we don’t know about these tiny stars, so as we search for Earthlike planets we also want to get a better census of their overall planet population. That way, we can better understand how those planets originally formed.
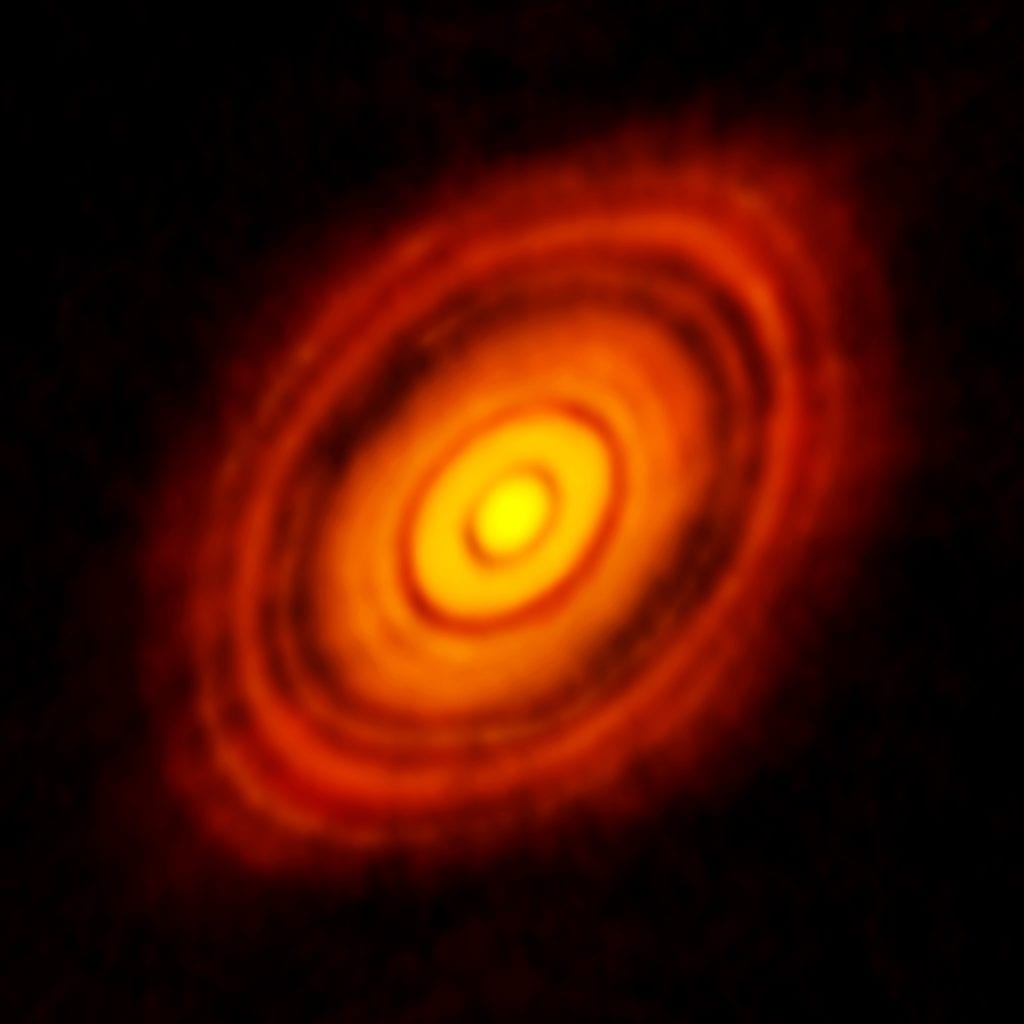
A protoplanetary disk orbiting the young star HL Tau. The dark gaps in the disk may be caused by newly-formed planets collecting material. Image credit: Ralph Bennett – ALMA (ESO/NAOJ/NRAO)
Stars originally form from large clouds of gas and dust in interstellar space. The cloud collapses to form the star, and a small fraction of the original cloud remains as a disk of material orbiting the newborn star. Over the first few million years of the system’s life, the gas and dust coalesces into planets. Smaller stars tend to have smaller disks, so our current theories of planet formation predict that tiny M dwarf stars should form fewer planets, and in particular should form fewer large, gaseous planets like those found in our outer Solar system. Earlier surveys for planets orbiting M stars indeed found fewer giant planets. But to ensure we have a firm grasp on the physics of planet formation around all stars, we need to study more targets, and for longer periods of time.
In our HPF survey for M dwarf exoplanets, we have discovered a Neptune-sized exoplanet orbiting very close to an extremely low-mass star, LHS 3154. This discovery comes as a surprise in light of the aforementioned planet formation theories, and forces us to consider modifications to the theory at the low-mass end of the stellar sequence.
LHS 3154
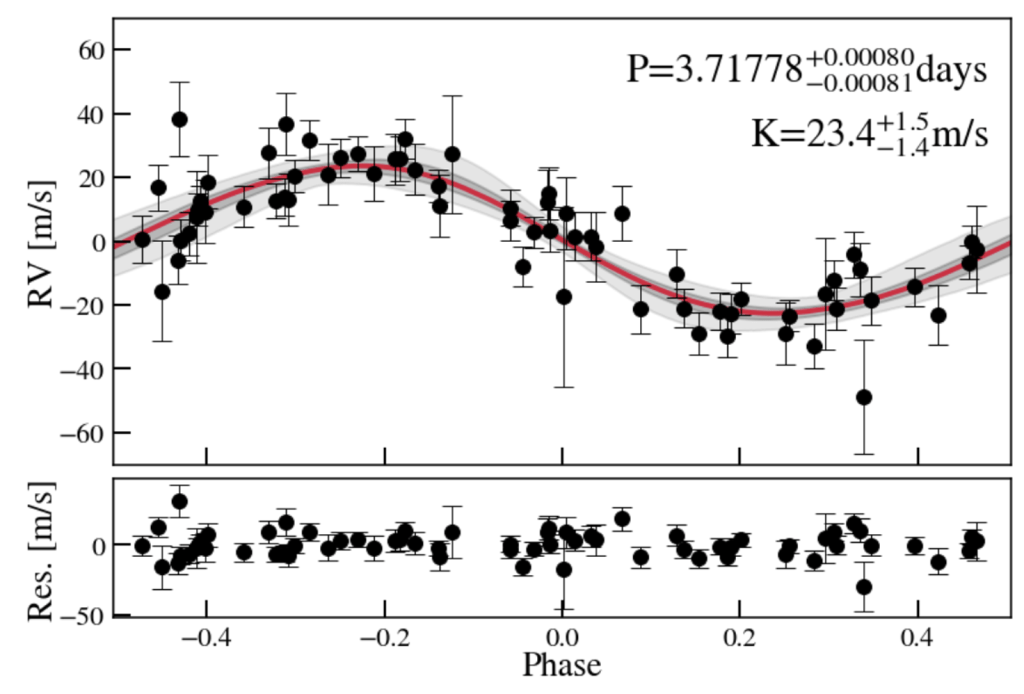
HPF radial velocity measurements of LHS 3154, mapped to the orbital phase of its Neptune-sized planet. The red curve shows the best fit model, and the grey curves show 1- and 3-sigma confidence intervals. ‘P’ shows the best fit orbital period in days and its uncertainties, and K shows the semi-amplitude in meters per second.
The star LHS 3154 is a target of the HPF survey for exoplanets orbiting M dwarf stars near the Solar system. It has a mass just 11 percent that of the Sun, and puts out only 0.1% as much energy as the Sun. A star must have at least 8 percent the mass of the Sun to sustain nuclear fusion in its core, so LHS 3154 is among the smallest stars we could expect to find. At such a small size, we place LHS 3154 into a category of objects (somewhat unimaginatively) called “very low-mass stars,” or VLM stars.
VLM stars such as LHS 3154 have very little history in exoplanet surveys, as they are too faint and red for optical spectrometers. Their energy spectrum peaks well into the near-infrared, making them ideal targets for HPF. We started surveying the star in early 2020, and quickly noticed the star exhibiting a characteristic wobble like we would expect if it hosted one or more planets. We watched it carefully, as VLM stars frequently exhibit signals from starspots in their atmospheres that can look a lot like planets. But over time, it became clear that the signal remained constant, and was not connected to the star’s magnetic activity. We had a real planet!
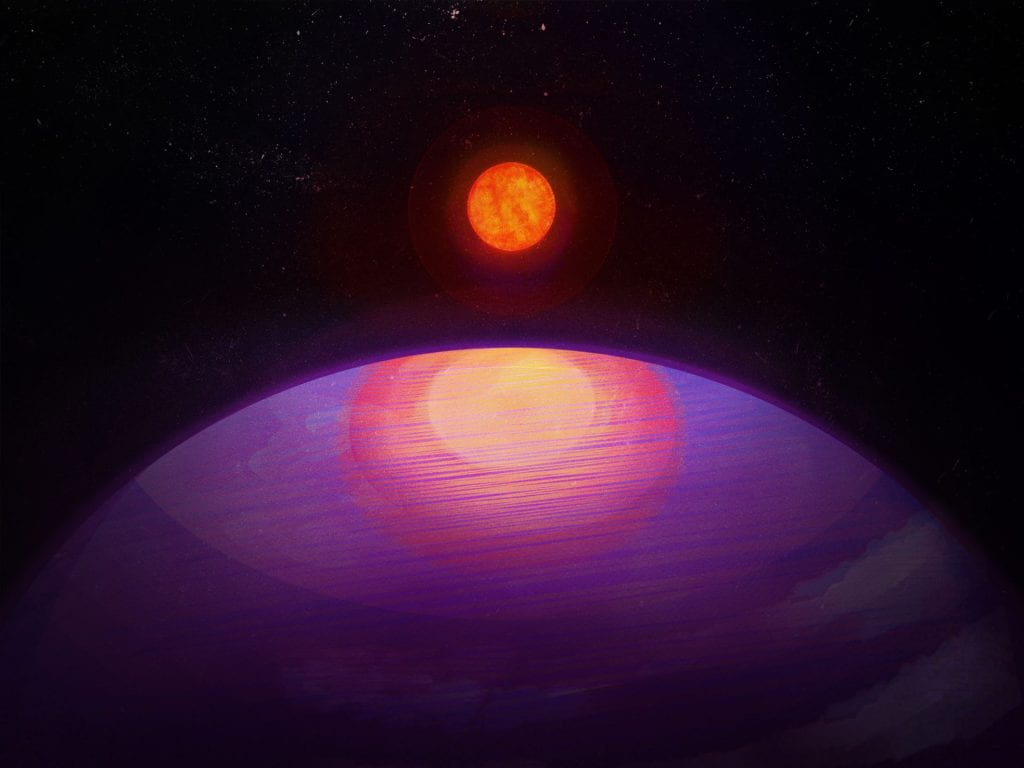
Artistic rendering of the possible view from LHS 3154b towards its low-mass host star. Given its large mass, LHS 3154b likely has a Neptune-like composition. Image credit: Thomas Klimek, Penn State
A Planet Out of Place
M dwarf stars are known to host plenty of planets, but they tend to be on the smaller side. Again, this is consistent with what we understand about star and planet formation; the protoplanetary disks we have measured around young M stars typically only have enough solid material to form a few rocky planets the size of Earth or so. The planet we found orbiting LHS 3154–named LHS 3154b–does not meet this description: it has a mass similar to that of Neptune, more than 13 times the mass of Earth. Furthermore, it follows a tight orbit around the star, at just 2 percent the separation between Earth and the Sun.
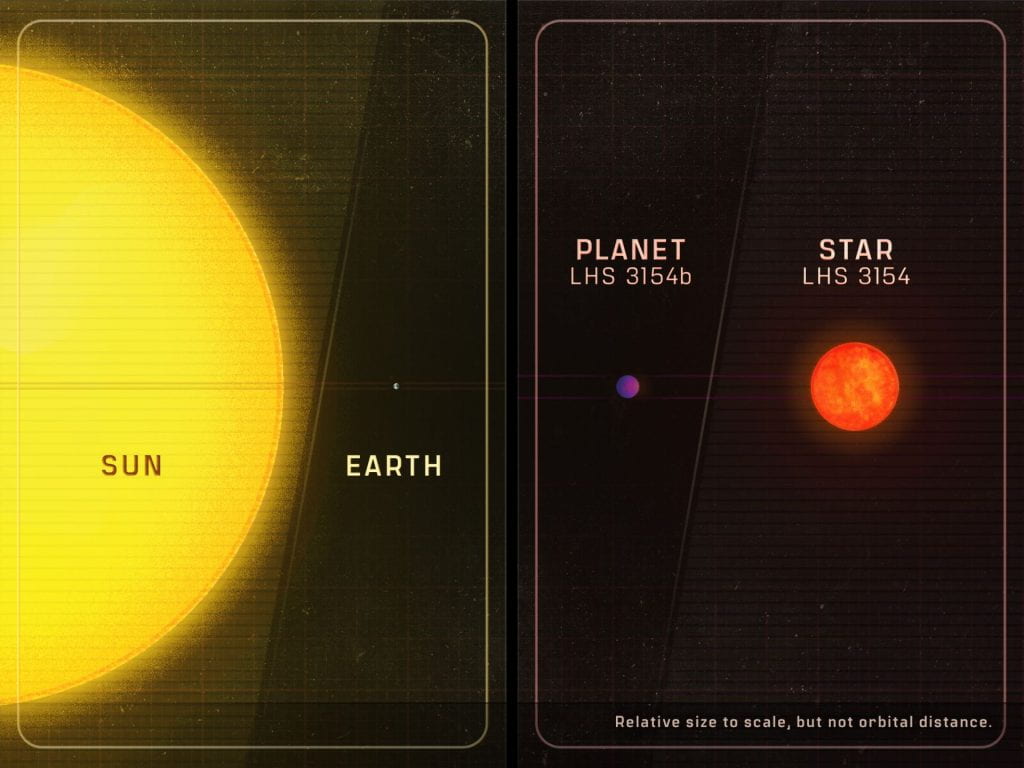
Relative sizes of the star LHS 3154 and its planet, with the Sun/Earth system for comparison. The large size of the planet LHS 3154b relative to its host star is unusual among known exoplanets. Image credit: Thomis Klimek, Penn State
None of our current theories of planet formation predict such a planet should be found around a VLM star! We performed computer models of the core accretion process, where small bits of solid material in a protoplanetary disk collide and grow into planet-sized objects. While LHS 3154 is too old to still have a protoplanetary disk, we can make some reasonable estimates of what properties it once had, and simulate the planets it would be likely to form. Even when making optimistic assumptions about the geometry of the disk, and the amount of solid material it contained, our models rarely produced planets as massive as LHS 3154b on such close-in orbits.
There are a few possibilities for what’s going on here. Either LHS 3154b is an extreme outlier in the Galactic planet population, or our models of planet formation are incomplete. The only way to determine which is to keep searching for planets. If we never find any more planets like LHS 3154b, then it may just be the rare extreme outcome of a well-understood process. But if we find more like it, we’ll have to revise our models to account for their formation.
Interestingly, despite LHS 3154b’s extreme proximity to its host star, the star’s faintness means the planet actually orbits near the inner edge of the system’s liquid-water Habitable Zone (HZ). We don’t expect that the planet is habitable for Earthlike life; it is massive enough that any lifeform would experience deadly atmospheric pressure long before it reached the planet’s surface. But this discovery still holds important implications for habitable planets in the system. Having a massive planet so close to the habitable zone potentially means any other planets in the HZ would be gravitationally destabilized by LHS 3154b. So this might not be the best system to search for life!
Find Out More
Our study of LHS 3154 is described in a research article led by HPF Team member Gudmundur Stefansson, which was recently published in Science. Check it out for all the details!