Introduction: Transmission Spectroscopy
As we have covered in previous posts, HPF was designed and built for the goal of detecting exoplanets through the Doppler motion of their host stars. Of course, there are other astrophysics experiments that can take advantage of HPF! There are plenty of applications for a stable, high-resolution near-infrared spectrometer. In today’s post, we will discuss one such application that allows us to learn more about nearby exoplanets: transmission spectroscopy.
Normally, it is extremely difficult to learn anything about the atmosphere of an exoplanet. The planet itself is lost in the glare of its host star, and for a planet like Earth, the atmosphere is a tiny component of its overall radius–if Earth were the size of an apple, the atmosphere would only be as thick as a normal apple’s skin. One special situation in which we can catch a glimpse of an exoplanet’s atmosphere is when the planet transits (or eclipses) its star. When a transit occurs, a tiny (but measurable!) fraction of the star’s light will filter through the planet’s atmosphere, where atoms and molecules in that atmosphere will absorb light at very specific wavelengths. Thus, by comparing the normal spectrum of the star with the spectrum as observed during transit, we can determine which chemical species are absorbing light in the planet’s atmosphere. With the right contextual information, we may also be able to probe properties like the atmospheric temperature, pressure, and more.
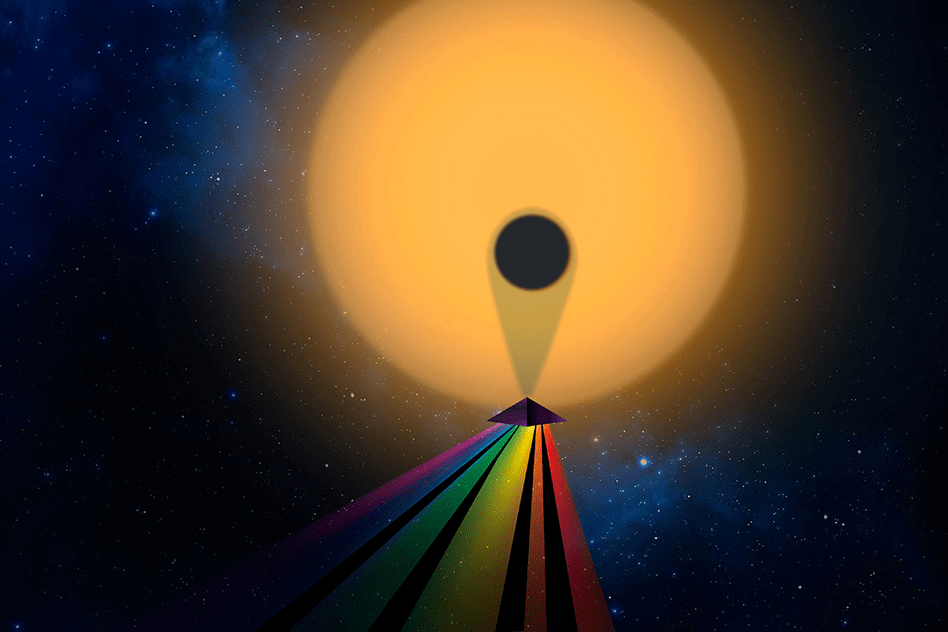
Transmission spectroscopy examines starlight filtered through an exoplanet’s atmosphere to determine the composition and other properties of the planet’s atmosphere (Image credit: Christine Daniloff/MIT, Julien de Wit).
Planets orbiting very close to their host stars get so hot that their atmospheres begin to escape into space, trailing behind the planet like a giant comet tail. Recent simulations of these escaping atmospheres suggest that absorption of near-infrared light by helium atoms should create a large signal for transmission spectroscopy. The wavelength of this helium absorption is right in the middle of HPF’s waveband, so as an early science program for HPF, we attempted to detect this effect for an exoplanet transiting an M dwarf star.
The target: GJ 3470b
We have mentioned the M dwarf star GJ 3470 on this blog before–it was actually the star chosen for HPF’s first light image. The star hosts a planet about the size of Neptune orbiting very close, which is the best type of planet for atmospheric characterization. Other studies have already suggested that the planet should have a blue sky! Based on what was already known about the planet’s size, density, and stellar radiation exposure, we predicted it should exhibit significant absorption from helium. So we set out to determine whether HPF could measure it.
The experiment
When you need a planet to be in front of its star in order to make a measurement, the timing is crucial. Careful planning is needed to observe the star at a time when a transit occurs at night from your observatory, and the weather has to be good. In the case of GJ 3470b, we had to observe three such events in order to develop enough signal from the helium absorption. In the meantime, we also observed the star when the planet wasn’t transiting, in order to compare the in- and out-of-transit cases. All said, it took us about 5 months to get all of the observations required to do this experiment.
So did we see helium absorption?
To date, GJ 3470b is the smallest planet for which atmospheric helium absorption has been measured. As a result, the helium signal is relatively small, and we had to be careful with how we merged all the observations into a single spectrum. We also had to convince ourselves that the signal was not created by changes in the HPF instrument, or in Earth’s atmosphere.
An additional complication comes from the fact that the atmosphere of GJ 3470b is so hot that the temperature causes helium atoms to fly out of its atmosphere into space at high velocity, in a process known as a Parker wind. This motion of the helium gas distorts the absorption feature we would otherwise expect to see, so we had to carefully model the effects of the Parker wind in order to make sure our data were consistent with the all of the physics involved in the interaction of the planet’s atmosphere with the intense stellar radiation.
After accounting for all of these effects, we determined that the HPF spectra show evidence for helium absorption caused by the atmosphere of the planet. In the image below, we show the comparison between the spectrum of the star with and without the planet passing in front. The spectrum shows a little more absorption at the wavelength of the helium feature during transit, in approximately the shape we expect from the wind-shifted motions of the atmosphere. The signal is weak, but our careful analysis of the data in combination with the wind model suggests it is real. Our detection is also supported by observations of the same planet by another team using the CARMENES spectrometer.
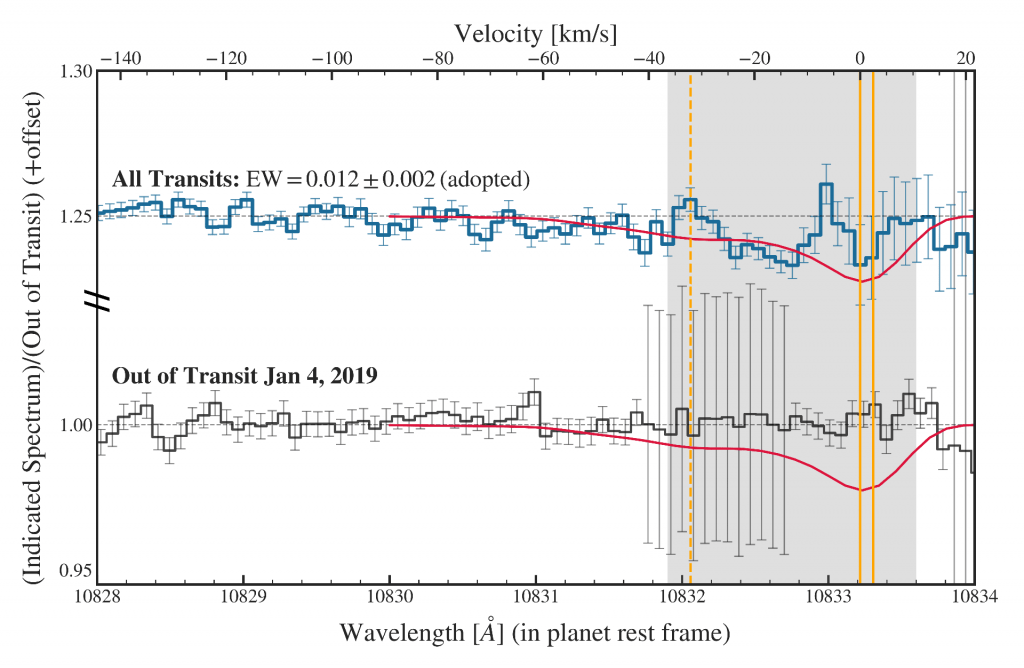
Spectra of GJ 3470 during (top) and outside of (bottom) the transit of its planet. The red curve shows our model of the expected absorption from helium in the planet’s atmosphere. Only during transits does the star’s spectrum show the expected absorption!
In short: the technique works! This detection is an enticing preview of what’s possible in terms of atmospheric characterization of exoplanets using HPF.
Curious case of blue-shifted broad absorption in GJ3470b
While infrared helium absorption has been detected earlier in the atmospheres of larger planets, the most puzzling aspect of our new detection in the first warm Neptune was its unusually broad absorption profile. This is especially true on the blue-wavelength side (leftwards in the image above), since the red side of the profile has more noise due to contamination from Earth’s atmosphere.
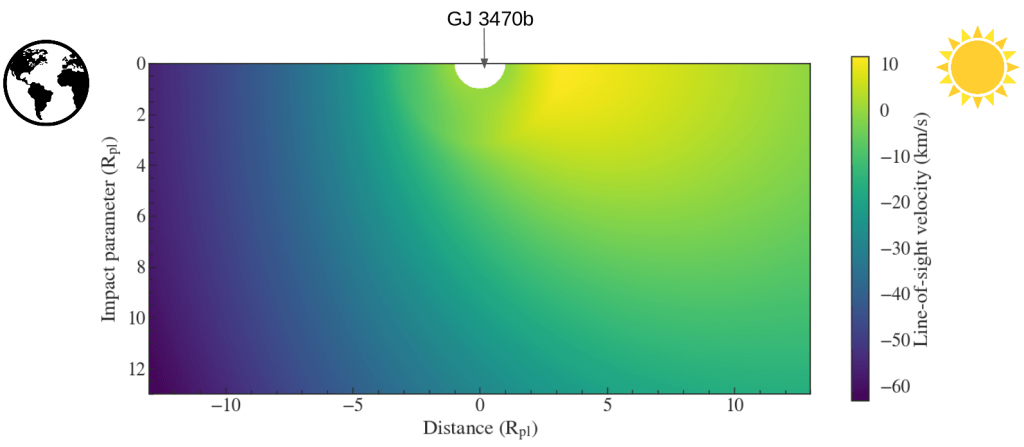
The line-of-sight velocity field of upper atmosphere around GJ 3470b. An Earth-based observer is towards the left side of the plot, and the host star GJ 3470 is towards the right side. Bluer colors indicate more rapid motions towards Earth.
The reason turned out to be simple orbital dynamics. The orbit of GJ 3470b is not perfectly circular, and so its motion along the line connecting the star and Earth (the so-called “line of sight velocity”) is actually slowing down during the transit. Just like a slingshot, helium atoms escaping the gravitational pull of the planet continue to move towards us at the velocity they had the moment they escaped from the planet. Once you incorporate this velocity change into the modeled absorption profile from the helium atoms, it matches well with the observation!
What did we learn about the planet?
These measurements allowed us to estimate properties like the density, temperature, and velocity of helium atoms in the planet’s atmosphere. In the image below, we have actually mapped out the distribution of helium surrounding the planet itself.
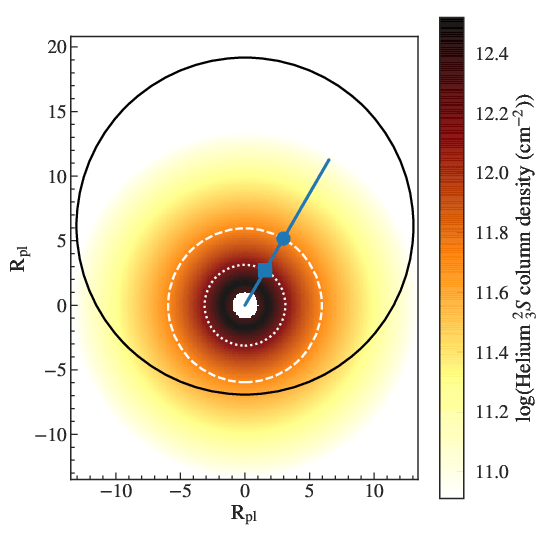
Scale model of the star GJ 3470 (large black circle) with its planet (inner white circle) in transit. The red-yellow color scale indicates the density of helium atoms surrounding the planet, based on our transmission spectrum.
With powerful instruments and enough observing time, we can learn a lot more about a planet’s atmosphere. Similar observations can reveal different chemical species, probe deeper into a planet’s atmosphere, and monitor changes over time. Eventually, we will use this technique to look for changes in planetary atmospheres that could be caused by life! So while this first step for HPF is exciting, it is only scratching the surface of an aspect of exoplanet study that will become increasingly important in the coming years.
Where can I find out more?
Details of this experiment were recently published in the AAS Journals in an article led by HPF team member Joe Ninan. We encourage you to go there for more information!